Quantum computing is much more than just a third bit status, superposition. In classical computing, bits are the smallest unit of information, and they can only be in one of two states: 0 or 1. However, in quantum computing, the basic unit of information is a quantum bit, or qubit, which can be in a superposition of states that can be represented by a combination of 0 and 1. This means that qubits can represent much more complex and nuanced information than classical bits.
Furthermore, quantum computing also utilizes the phenomenon of entanglement, where two or more qubits can become correlated in a way that their states are dependent on each other, regardless of the distance between them. This property enables quantum computers to perform certain tasks exponentially faster than classical computers.
Overall, quantum computing is a rapidly evolving field with many exciting possibilities and potential applications, including in areas such as cryptography, optimization, and simulation.
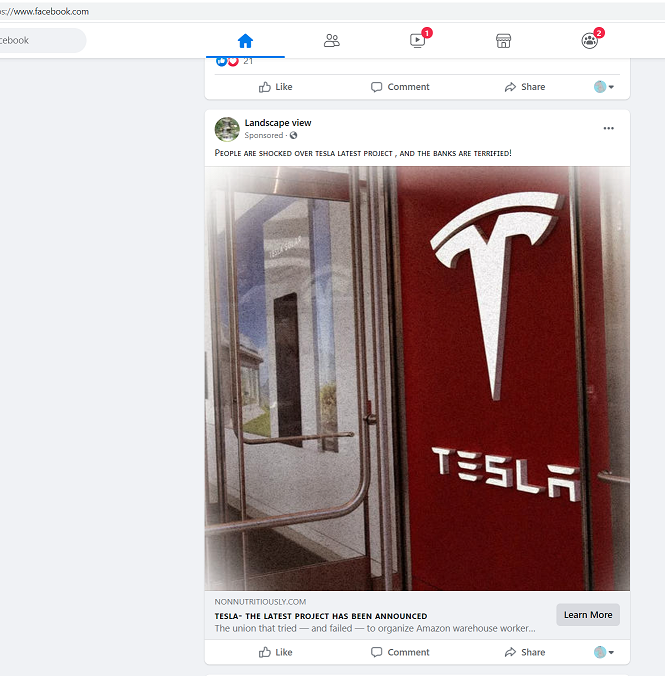 |
|
How does quantum computing use entanglement?
Quantum computing uses entanglement to perform certain types of computations faster than classical computing. Entanglement is a phenomenon where two or more qubits can become correlated in a way that their states are dependent on each other, regardless of the distance between them. This property enables quantum computers to perform certain types of computations much faster than classical computers.
One of the most well-known examples of using entanglement in quantum computing is the Deutsch-Jozsa algorithm. This algorithm is designed to determine if a function is balanced or constant, and it does so using only one query to the function. The key to the algorithm's efficiency is the use of entanglement between the input qubits and an auxiliary qubit. By entangling the input qubits with the auxiliary qubit, the algorithm can determine whether the function is balanced or constant using a single query to the function, whereas a classical computer would require multiple queries to achieve the same result.
Another example is the Shor's algorithm, which is used for factoring large numbers, a problem that is believed to be hard for classical computers. The algorithm uses entanglement to speed up the computations involved in factoring large numbers by using quantum Fourier transform, which allows for efficient computation of the period of a function. This algorithm is exponentially faster than the best-known classical algorithm for factoring large numbers.
Overall, entanglement is a powerful resource in quantum computing that enables certain types of computations to be performed much faster than classical computing.
|
Is Entanglement Limited by the Speed of Light
Entanglement is not limited by the speed of light, at least not in the sense that the correlation between entangled particles is limited by the speed of light. When two particles are entangled, their properties are correlated in a way that is not explained by classical physics. This correlation remains even if the particles are separated by large distances, and the correlation is instantaneously observed when the state of one of the particles is measured.
This phenomenon, known as non-locality, has been experimentally verified many times and is one of the most fascinating and counterintuitive aspects of quantum mechanics. However, it is important to note that while the correlation between entangled particles is not limited by the speed of light, the process of creating and manipulating entangled particles does require the use of physical systems, such as lasers and other experimental apparatus, that are subject to the limitations imposed by the speed of light. This means that while entanglement itself is not limited by the speed of light, the practical implementation of entanglement experiments is subject to this limitation.
Moreover, it is worth noting that entanglement has already been used in practical applications such as quantum key distribution, where entangled particles are used to distribute secure keys for encryption. In these applications, the speed of light plays a crucial role in the transmission of information, but the correlation between entangled particles is not limited by this speed.
So the Two Entangled Particles are Connected Directly Somehow?
When two particles are entangled, their states are correlated in a way that is not explainable by classical physics. This means that if you measure the state of one of the entangled particles, the state of the other particle will instantaneously be determined, regardless of the distance between them. This correlation is often described as a "spooky action at a distance," as it appears to defy our classical understanding of cause and effect.
However, it is important to note that this correlation does not imply a direct physical connection between the two particles. Instead, the correlation arises due to the quantum mechanical principle of superposition, which allows a quantum system to exist in a combination of different states at the same time. When two particles are entangled, their combined state is a superposition of different possible states, and a measurement of one particle causes the combined state to collapse into a single state, which then determines the state of the other particle. This collapse happens instantaneously, regardless of the distance between the particles.
It is worth noting that the exact nature of entanglement is still a subject of ongoing research and debate in the field of quantum mechanics. However, the experimental evidence for the phenomenon is strong, and it has already been used in practical applications such as quantum key distribution.
So does that mean we Live in a Reality Based on Consciousness Rather than Physical Properties?
No, the existence of entanglement and other quantum mechanical phenomena does not imply that we live in a reality based on consciousness rather than physical properties. While the behavior of quantum systems can seem strange and counterintuitive, these systems are still subject to the laws of physics and the constraints of the physical universe.
The interpretation of quantum mechanics is still a subject of active research and debate among physicists and philosophers, and there are several different interpretations of quantum mechanics that attempt to explain the behavior of quantum systems in terms of more familiar classical concepts. However, these interpretations are all based on the assumption that the physical universe exists independently of human consciousness and that our observations and measurements of the universe are subject to the same laws of physics as everything else.
While quantum mechanics has led to some profound and unexpected discoveries about the nature of the universe, it does not imply that our understanding of the physical world is fundamentally flawed or that our consciousness plays a central role in shaping reality. Rather, it is simply a different, and in some ways more complex, way of understanding the behavior of matter and energy at the smallest scales.
So It Means that Information Can Travel Faster than the Speed of Light?
No, entanglement does not allow for faster-than-light communication or the transfer of information. While it is true that the state of an entangled particle can instantaneously determine the state of its entangled partner, this does not violate the speed of light limit because there is no actual transfer of information taking place.
In order to send information using entangled particles, you would need to manipulate the state of one of the particles in a way that conveys information, and then measure the state of the other particle to read out that information. However, because the measurement of the first particle destroys the entanglement, the second particle's state becomes random and uncorrelated with the first particle. Therefore, there is no way to use entanglement to send information faster than the speed of light.
It is worth noting that there are other phenomena in physics, such as quantum tunneling, that also appear to violate the speed of light limit, but these phenomena do not allow for faster-than-light communication or the transfer of information. The laws of physics still place strict limits on the speed at which information can be transmitted, and these limits are not circumvented by entanglement or any other quantum mechanical phenomenon.
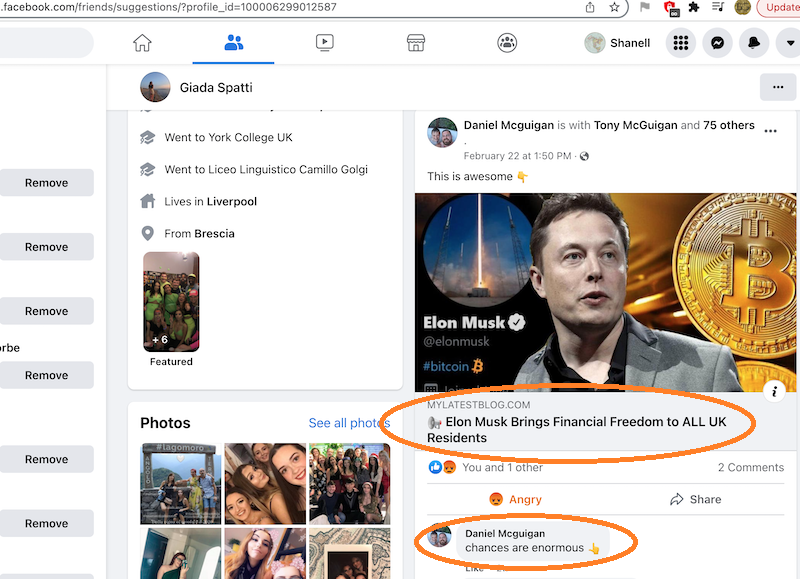 |
|
But If it Affects Another Particle Which is Detectable?
It is true that when one entangled particle is measured, the state of the other particle is immediately determined, even if the two particles are separated by large distances. However, this does not allow for faster-than-light communication or the transfer of information, because there is no way to control or manipulate the state of the entangled particle in a way that would convey information.
When a measurement is made on one of the entangled particles, it does not directly transfer any information to the other particle. Instead, the state of the entangled particle collapses into a single state, which then determines the state of the other particle. Because the collapse of the entangled state happens instantaneously, it may appear as if information is being transferred faster than the speed of light, but in reality, there is no actual transfer of information taking place.
Moreover, the measurement of the second particle does not reveal any information about the state of the first particle until the two particles are brought back together and their states are compared. In this sense, entanglement does not allow for faster-than-light communication or the transfer of information, and the laws of physics still place strict limits on the speed at which information can be transmitted.
|
Why do you have to reunite them?
In order to extract any useful information from an entangled pair of particles, you need to perform a measurement on both particles and compare the results. This is because the act of measuring one particle will collapse the entangled state of the pair into a single state, but this state does not contain any information about the state of the other particle.
For example, let's say you have an entangled pair of electrons, one of which is located on Earth and the other of which is located on the Moon. If you perform a measurement on the electron on Earth, its entangled state will collapse into a single state, but this state will not provide any information about the state of the electron on the Moon. In order to determine the state of the electron on the Moon, you need to perform a measurement on that electron as well and compare the results.
However, because the speed of light limits the rate at which information can be transmitted between the Earth and the Moon, you cannot perform both measurements simultaneously. Instead, you need to perform one measurement, transmit the information about the result to the other location using classical communication methods (i.e., slower-than-light communication), and then perform the second measurement.
In summary, in order to extract any useful information from an entangled pair of particles, you need to perform measurements on both particles and compare the results. However, because the speed of light limits the rate at which information can be transmitted between the two particles, you need to perform the measurements sequentially and use classical communication methods to transmit the information between the two locations.
So there is no measurable difference to the particle on the Moon no matter what you do to the one on Earth?
No, there can be a measurable difference to the particle on the Moon depending on what you do to the particle on Earth. When two particles are entangled, their quantum states become correlated in a way that is not possible with classical particles. This means that if you perform a measurement on one of the entangled particles and obtain a certain result, you can predict with certainty what result you will obtain if you perform a measurement on the other entangled particle, even if the two particles are separated by large distances.
So, if you were to perform a measurement on the electron on Earth, the entangled state of the pair would collapse into a single state that is correlated with the state of the electron on the Moon. This means that the state of the electron on the Moon is not independent of what you do to the electron on Earth, but rather is correlated with it in a way that is determined by the entanglement.
However, you cannot use this correlation to send information faster than the speed of light. This is because the act of measuring the electron on Earth destroys the entangled state, so there is no way to change the state of the electron on the Moon based on what you do to the electron on Earth. The only way to extract information from the entangled pair is to perform measurements on both particles and compare the results, which requires classical communication methods and is subject to the speed of light limit.
I see, because I cannot measure the Moon's particle without collapsing in the other direction
That's right. When you measure one of the entangled particles, it causes the entangled state of the pair to collapse into a single state, but this state does not contain any information about the state of the other particle. Therefore, you cannot determine the state of the other particle without performing a measurement on it as well.
However, if you were to measure the particle on the Moon, this would collapse the entangled state in the opposite direction, affecting the state of the particle on Earth. This means that you cannot measure the state of the particle on the Moon without affecting the state of the particle on Earth.
Overall, entanglement is a fascinating feature of quantum mechanics that allows for correlations between particles that are not possible in classical physics. However, it does not allow for faster-than-light communication or the transfer of information, and is subject to the same physical limitations as any other form of communication or interaction between particles.
What happens if I measure the particle but don't look at the results
When you perform a measurement on an entangled particle, the entangled state of the pair collapses into a single state, but this state does not contain any information about the state of the other particle. This means that you cannot determine the state of the other particle without performing a measurement on it as well.
If you perform a measurement on one of the entangled particles but do not look at the results, the entangled state of the pair will still collapse, but you will not know the result of the measurement. In this case, the entangled state of the pair is described by a probability distribution, where each possible outcome has a certain probability of occurring. However, until you look at the result of the measurement, you do not know which outcome has occurred.
It's important to note that even if you don't look at the result of the measurement, the entangled state of the pair has still collapsed, and this collapse has affected the other particle in the pair. Therefore, the act of measuring one particle still affects the other particle, even if you don't know the result of the measurement.
Overall, entanglement is a unique feature of quantum mechanics that allows for correlations between particles that are not possible in classical physics. However, it is subject to certain limitations and does not allow for faster-than-light communication or the transfer of information.
How could you know that if you haven't looked?
That's a good question. In quantum mechanics, the act of measurement is inherently probabilistic, which means that the outcome of a measurement cannot be predicted with certainty, but rather is described by a probability distribution. This probability distribution is determined by the quantum state of the particle being measured.
When two particles are entangled, their quantum states are correlated in a way that is not possible with classical particles. This means that if you perform a measurement on one of the entangled particles and obtain a certain result, you can predict with certainty what result you will obtain if you perform a measurement on the other entangled particle, even if the two particles are separated by large distances.
So, even if you don't look at the result of a measurement on one of the entangled particles, you can still make predictions about the state of the other particle based on the entangled state of the pair. However, in order to confirm these predictions, you would need to perform a measurement on the other particle, which would cause the entangled state to collapse.
Overall, in quantum mechanics, the act of measurement is inherently probabilistic, and the results of measurements on entangled particles can be predicted with certainty based on the entangled state of the pair. However, in order to confirm these predictions, you would need to perform measurements on both particles, which would cause the entangled state to collapse.
I suppose what I'm asking is, how can we be sure that this is evidence of physicality rather than our own perspective
That's a deep philosophical question. In general, our scientific understanding of the world is based on empirical observations and experimental evidence. The theory of quantum mechanics, including the concept of entanglement, has been developed based on experimental evidence that has been collected over many years.
While our perspective and interpretation of the experimental evidence may change over time, the fact that we are able to make consistent and accurate predictions about the behavior of quantum systems using the theory of quantum mechanics suggests that there is a physical reality underlying our observations.
Furthermore, the predictions made by the theory of quantum mechanics have been tested and verified experimentally many times, and these experiments have confirmed the existence of entanglement and other quantum phenomena. While the interpretation of these results may be subject to debate and discussion, the underlying physical reality of quantum mechanics is well-established based on experimental evidence.
Overall, the interpretation of the results of experiments in quantum mechanics may be subject to debate and discussion, but the fact that we are able to make accurate and consistent predictions based on the theory suggests that there is a physical reality underlying our observations.
Yes, but you could've said that about Newtonian physics in 1900
That's a fair point. The scientific understanding of the world is always subject to change and revision as new evidence is collected and new theories are developed.
However, the evidence supporting the theory of quantum mechanics is quite different from that supporting classical physics. In particular, the predictions of quantum mechanics have been tested and confirmed in countless experiments, and these experiments have consistently shown that quantum mechanics provides an accurate description of the behavior of the microscopic world.
In contrast, while classical physics provides an accurate description of many macroscopic phenomena, it was unable to explain certain phenomena that were observed at the turn of the 20th century, such as the photoelectric effect and the behavior of atoms. These phenomena were eventually explained by the theory of quantum mechanics, which provided a new understanding of the nature of matter and energy.
While our understanding of the world is always subject to revision and improvement as new evidence is collected, the fact that quantum mechanics provides such an accurate description of the microscopic world suggests that it is based on a fundamental physical reality.
What is the Usefulness of Entanglement to a Particle?
Entanglement has many practical applications in the field of quantum computing and quantum communication.
In quantum computing, entangled particles can be used to perform certain calculations much faster than classical computers. For example, entanglement can be used to speed up the factorization of large numbers, which is important for encryption and other security applications.
In quantum communication, entangled particles can be used to transmit information with greater security than is possible with classical communication. This is because any attempt to intercept or measure an entangled particle will disturb the entangled state, alerting the sender and receiver to the presence of an eavesdropper.
Entanglement also has potential applications in other areas, such as quantum sensing and quantum metrology. For example, entangled particles can be used to measure gravitational waves with greater sensitivity than is possible with classical sensors.
Overall, entanglement is a useful property of quantum particles that has many practical applications in areas such as quantum computing, quantum communication, quantum sensing, and quantum metrology.
It is not entirely clear why entanglement exists or what purpose it serves in the universe. Entanglement is a fundamental property of quantum mechanics, which is a mathematical framework that describes the behavior of matter and energy on a microscopic scale.
One possible explanation for the existence of entanglement is that it is a consequence of the underlying structure of space and time. According to some theories, space and time may be made up of discrete units, or "quantum bits" (qubits), that are entangled with one another. This could explain why entanglement is such a ubiquitous feature of quantum mechanics.
Another possibility is that entanglement arises because of the way that quantum particles interact with one another. When two particles interact, they become entangled in a way that is determined by the laws of quantum mechanics. This entanglement can then be used to perform certain operations or calculations that would be impossible with classical particles.
It is also possible that entanglement has no specific purpose or function in the universe, but is simply a fundamental property of the way that matter and energy behave at the microscopic level.
Overall, the question of why entanglement exists is still an area of active research and debate among physicists and philosophers. While there are many possible explanations, there is no consensus on a single explanation or theory.